FUEL & FUEL SYSTEM MICROBIOLOGY PART 19 – PREVENTING MICROBIAL DAMAGE TO FUEL SYSTEMS PART 2; WATER
Disclaimer:
As in my previous post, I’ll open this post with a disclaimer. Microbes are ubiquitous. There are extraordinarily few habitats on earth where thriving, microbial communities have not been detected. In practical terms, this means that it is unlikely that operators will ever have a completely sterile fuel system or that they will reduce their fuel system biodeterioration risk to zero. Biodeterioration can still occur in the best maintained fuel systems. However, the risk of it occurring in an inadequately maintained system is much more likely.
No water means no bugs. Is it as easy as all that?
Best practice for keeping microbes from growing in fuel systems is to keep them dry. You’ll find this guidance in just about every consensus guidance document (for example ASTM D6469) and peer reviewed paper on the subject; including the ones I’ve written. Of course, there is a catch. In today’s post, I’ll write about why keeping fuel systems water-free is much easier said than done.
Water, water everywhere…
Water in fuel systems is typically present in three forms. Dissolved water is made up of individual water molecules and water droplets that are smaller than 1 m diameter (dia; 1 m = 0.00004 in) in fuel. Fuel containing only dissolve water appears to be transparent – no visible haze. The concentration of dissolved water that a fuel product can hold depends on temperature and product chemistry. Water solubility (also called the product’s water tolerance) increases with product temperature. For example, water solubility in E-10 gasoline increases from 0.2 % (by vol.) to 0.5 % as the product temperature increases from 0 C (32 F) to 20 C (68 F). For products typically sold at retail sites, water solubility is greatest in B-5 biodiesel. Water is least soluble in conventional gasoline.
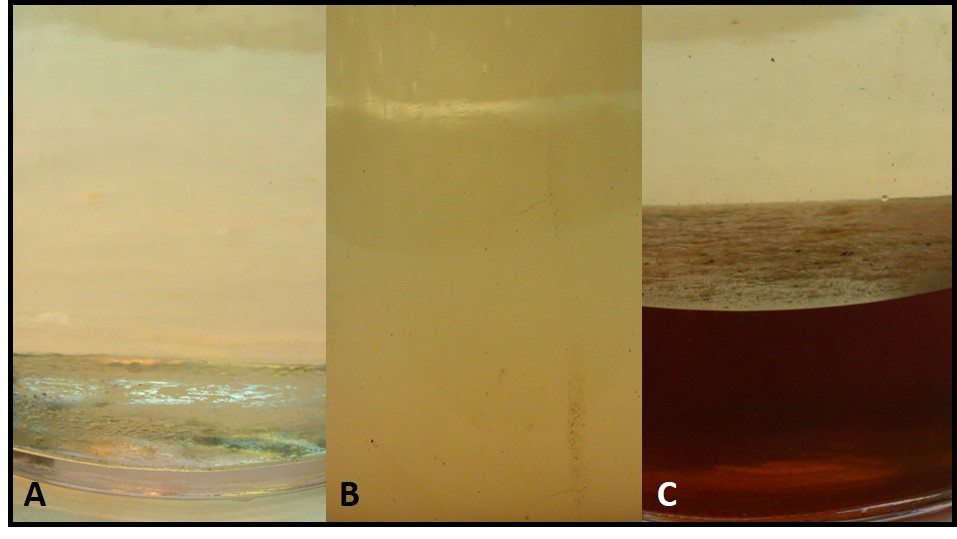
A – Clear and bright gasoline (ASTM D4176 haze rating: 1; B – gasoline with ASTM D4176 haze rating: 6; C – gasoline over bottoms-water; fuel’s ASTM D4176 haze rating: 3.
Once water concentration reaches its saturation limit, individual molecules join together to form dispersed water. Dispersed water droplet diameters range from 1 m dia to 10 m dia. Fuel haze (see ASTM D4176) increases with the number and size of dispersed water droplets. Droplets that are 10 m are heavy enough to settle out of the product and coalesce to form free water. Free-water can accumulate on tank walls, but is most commonly seen as bottoms water. Figure 1 shows three 87 octane gasoline fuel samples: figure 1A has < 0.1 % water (by vol.), figure 1B has an ASTM D4176 haze rating of 6 (i.e., with dispersed water), and figure 1C shows haze rating 3 fuel over free water.
Ethanol-blended gasoline (E-10)
There was a time when competent fuel chemists argued that phase-separation (i.e.: accumulation of bottoms-water) would never happen in E-10 tanks. They believed that because water was more soluble in E-10 than in E-0, there would never be sufficient water accumulation to drive the fuel-water split. The only problem is that they were wrong. Others argued that even if phase-separation did occur, the water-phase would be approximately 60% ethanol. Everyone (except microbes) knows that 60 % ethanol is a good disinfectant. Microbes couldn’t possibly grow in bottoms-water under E-10; except that they do. Most commonly, I do not detect active microbes in E-10 bottoms-water. On occasion, I do. Others have also reported detecting microbes in E-10 bottoms-water. We are not sure what’s going on (i.e., why the bugs are killed by the ethanol), but it is no doubt interesting. Bottom-line: do not assume that underground storage tanks (USTs) containing E-10 do not have bottoms-water or that bottoms-water under E-10 is microbe-free.
Implications
Assume that most UST have some free water. Here’s why:
- As explained above, depending on the fuel grade, good-quality quality (i.e., fit-for-use) product can as much as 0.5 % dissolved water.
- It is reasonable to assume that in a fuel carrying 0.01 % (i.e., 100 ppm) water, approximately 10 % of that water will separate from solution while product is in a UST. That 10 % of 100 ppm translates to 10 gal per million gal (a retail site that receives a 7,000-gal delivery every two to three days receives approximately 1 million gallons per year).
- Based on the previous bullet, approximately 10 gal of water will accumulate, if no other water enters the tank. Figure 2 illustrates this scenario. It shows three-years’ water accumulation in a 10,000 gal (8 ft diameter x 27 ft long underground storage tank; 10 gal = 0.3 in to 0.5 in water).
Figure 2. Underground storage tank (UST); 10,000 gal capacity, with 1 in (30 gal) water.
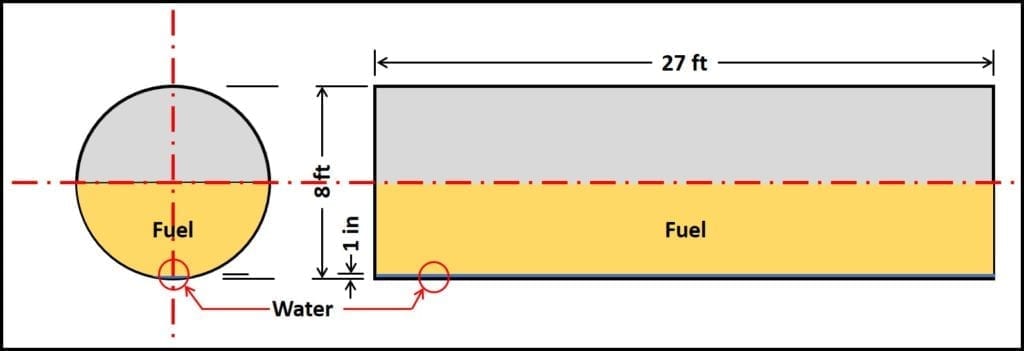
End and side view of UST that is approximately half filled with product. 1 in of water is approximately 30 gal; barely visible in this schematic. If water does not enter UST by any other means, it can take three years for this volume to accumulate.
End and side view of UST that is approximately half filled with product. 1 in of water 30 gal; barely visible in this schematic. If water does not enter UST by any other means, it can take three years for this volume to accumulate.
Using water-paste on a sounding stick, it should be easy to detect bottoms-water long before 1 in of water has accumulated. In fact, many companies specify that UST should be dewatered whenever 0.5 in or (10 gal to 15 gal) more water is detected. This guidance is based on two key assumptions:
- 1. USTs rest at the same angle at which they were installed: 1 in per 10 ft grade; with fill-line at low end (figure 3A).
- 2. UST floors (longitudinal, bottom dead-centerline) are straight (i.e. UST bottoms are flat). In the words of the song from Porgy and Bess: “taint necessarily so.”
There is a problem with these assumptions. USTs are installed on top of backfill. Despite best efforts to fully compact backfill before placing a UST, the tank’s weight – particularly after it has been filled – will cause additional backfill compaction (i.e., settling).
As illustrated in figures 3B and 3C, UST bottoms can settle flat or with the end opposite the fill-line (often the end with the submerged turbine pump – STP) lower than the fill-end. Tanks can also sag (lower in the center than at either end; figure 3D) hog (lower at both ends than in the center; figure 3E), or have numerous peaks and valleys along the longitudinal bottom centerline.
Figure 3. How USTs settle.
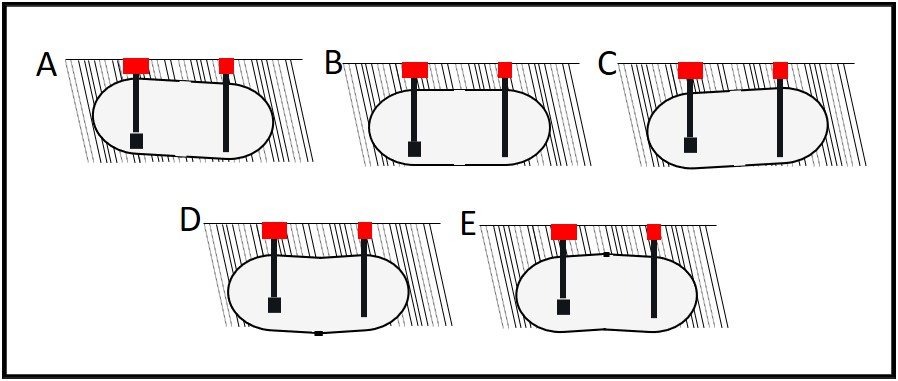
A – Typical, planned configuration: UST is lower at fill-end than at turbine end; B- UST is installed to lie flat; C – UST has settled so that turbine end is lower than fill end; D – UST has settled so that center is lower than ends; E – UST has settled so that ends are lower than center. All angles are exaggerated to illustrate settling issues.
Typically, the distance between peaks and valleys are measured in mm (1 mm = 0.04 in), so they are imperceptible to the naked eye. A 1 in per 10 ft incline is impossible to detect without using a bubble level. However, water will flow to low point(s). Long before free-water is detected, a UST is likely to have numerous, small pools of bottoms-water. Each is a great habitat for fuel-system microbes.
Can pools of 1 ounce (30 mL) water be habitats for microbes?
As illustrated in figure 4, to a bacterium a 2 mm deep pool of water (30 mL; 1 oz.) is like an large lake. In figure 4A, a 6 ft 6 in (2 m) tall man is standing at the foot of Mt. Kilimanjaro (19,700 ft – 6,000 m – tall). Figure 4B shows a bacterial cell (0.5 mm dia x 2 m long) “standing” at the bottom of a pool of water that is 2 mm (0.08 in) deep by 6 mm (0.24 in) wide. The relative height of Mt. Kilimanjaro’s peak over the man in figure 4A and the height of the pool of water over the bacterium in figure 4B is the same. In other words, from the perspective of microbes, traces of water that are undetectable to fuel system operators can be like large lakes to microbes; providing mini-habitats for millions of cells.
Figure 4. Scale: how 2 mm of water appears to a bacterial cell.
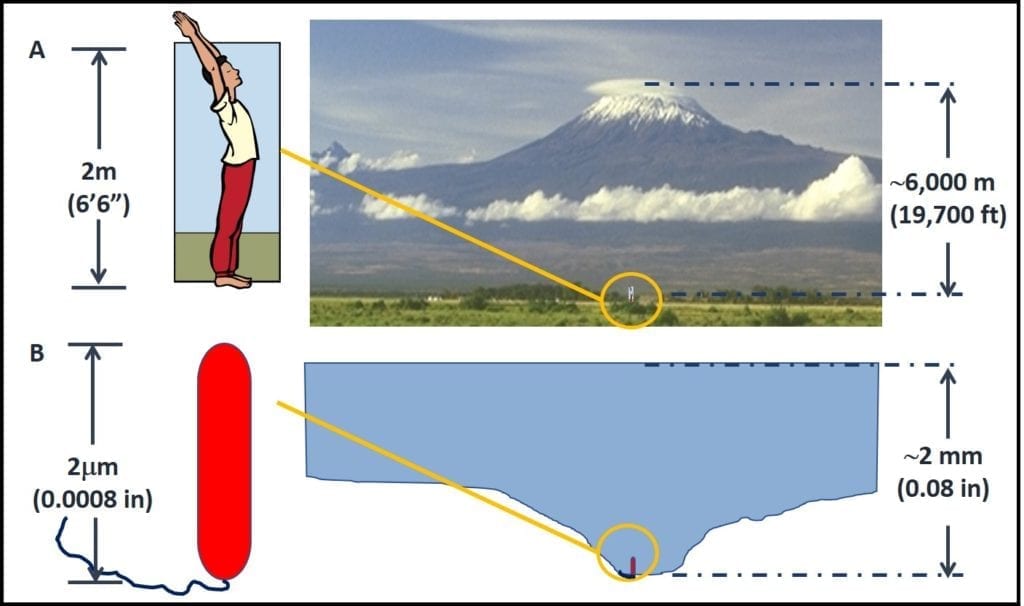
A – a 6’6” tall man standing at the base of Mt. Kilimanjaro; B- a bacterial cell “standing” in a pool of water that is approximately 2 mm deep; the ratios between the height of Mt. Kilimanjaro and the man in A, and between the depth of the pool of water and the bacterial cell in B are the approximately the same.
Summary:
In summary, water can be present in one or more of three forms in fuel systems:
- Dissolved
- Dispersed
- Free
It is much easier to prescribe keeping tanks water-free than it is to actually eliminate all water.
In my next blog, I’ll focus on options for minimizing water accumulation in fuel systems. In the meantime, if you have questions or comments about today’s post, please contact me at fredp@biodeterioration-control.com