MICROBIOLOGY FOR THE UNINITIATED – PART 1: WHAT ARE MICROORGANISMS AND WHY SHOULD WE CARE?
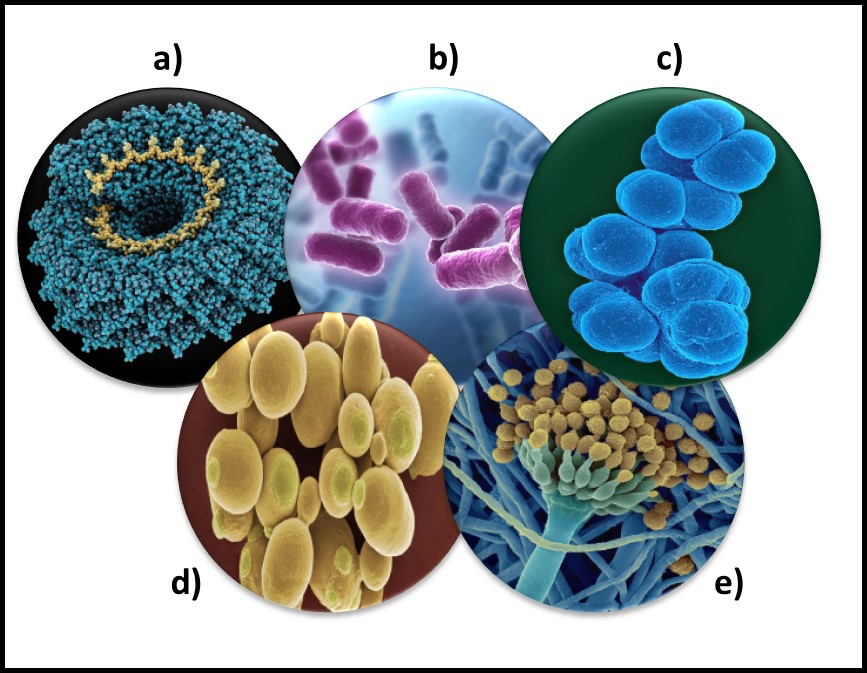
Microorganisms – a) virus; b) bacteria; c) archaea; d) yeast; d) mold.
Each year, on the first morning of STLE’s Metalworking Fluid Management Certificate Training Course, I ask attendees about their backgrounds. Invariably, the majority have at least undergraduate degrees in chemistry, engineering, or chemical engineering. However, of the more than 600 people who have taken the course since 2004, fewer than 50 have had a microbiology course.
Globally, biodeterioration – damage that organisms cause – has been estimated to range between $100 billion and $500 billion annually. Of this, 70 % to 80 % of biodeterioration damage is caused by microorganisms. Add to this an estimated $1 trillion cost due to infectious disease. On the flipside, biotechnology is a $500 billion industry that is based on microbiology. Just considering the economic impact microbe have on our lives, one might reasonably ask why so few people have received the most rudimentary education about microbes. Perhaps it’s the six syllables in microorganism (mic-ro-org-an-is-m) and microbiology that make the topic so intimidating. Still, given the role of microbes in disease, nature’s primary cycles, and biotechnology, one might think that every high school graduate would have learned something about microbiology. In this post and the next several to follow, I will do my best to demystify microbiology.
In this month’s article and those that follow, I’ll offer a very superficial overview of microbiology from an ecological and industrial perspective. There are numerous, excellent introductory microbiology textbooks. I don’t intend to provide any level of detail approaching that of a microbiology textbook. My intention is to help non-technical readers gain a fundamental appreciation for how microbes can affect their lives and their businesses.
Definition
Microorganisms (micro – meaning: small + organism – meaning: something having many related parts that function together as a whole) are living things that are too small to be seen by the naked eye. Microbiologists agree that bacteria, archaea, and some fungi (yeasts and molds) are microorganisms.
The status of virus is still a matter of debate. Viruses are essentially genetic material packaged in a protein coat. They don’t perform most of the functions that are used to define living beings. Thus most microbiologists do not consider viruses to be life forms. However, viruses reproduce by infecting cells and hijacking their victim’s (host’s) metabolic machinery to reproduce. Consequently, some microbiologists believe viruses should be classified as living things. Image (a) in the title figure shows a tobacco mosaic virus (TMV). The TMV virus is structurally complex, regardless of how we classify it. I included a virus in the in the figure because there are research groups investigating the use of viruses to control microbial contamination in industrial systems. I’ll return to this topic in a future post.
Abundance
One recent study, illustrated in Figure 1, estimated that microorganisms represent ~17% of Earth’s total biomass (estimated total biomass 550 gigatons of carbon – Gt C; 1Gt = 109 tons – and bacterial biomass ≈70 Gt)1. The contribution of bacteria to the Earth’s biomass is second only to that of plants. Microorganism biomass – including that of archaea, bacteria, fungi, protists, and viruses – account for ~93Gt.
A microbiome is the population of all microbes living in a specific ecosystem (fuel tank, cooling tower, human gut, etc.). Researchers investigating the human microbiome have estimated that the average human body has between 1x to 10x as many microbial cells as human cells. Moreover, it has been discovered that tissues, long thought to be microbe-free, have specific microbiomes that are likely to be essential for healthy tissue function (we have known about skin and gut microbes for nearly 170 years, but finding microbiomes specific to nearly every human tissue type (organs, muscle, etc.) was a surprise. Investigators have only scratched the surface of human microbiome research. We have little idea of how microbes interact with human cells and what roles they play in maintaining good health.
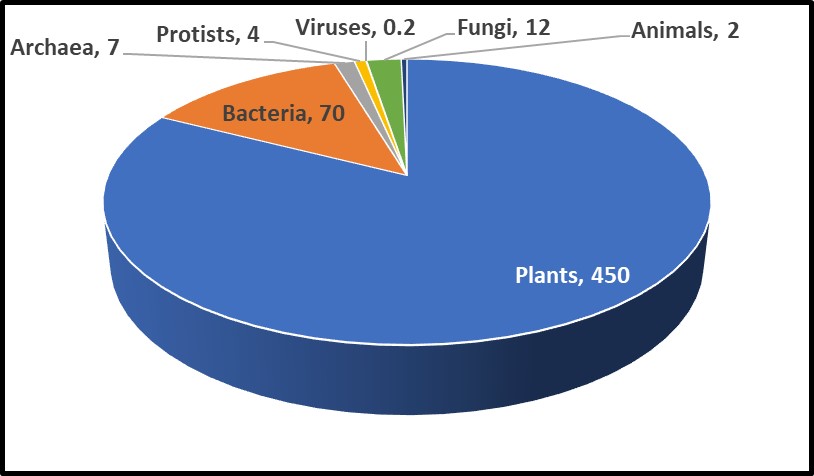
Fig 1. Relative abundance of Earth’s lifeforms, in Gt.
Diversity
As I will explain in future articles, the microbial world is remarkably diverse. The number of different types of bacteria has been estimated to range from hundreds of thousands to tens of millions, of which only a fraction of a percent has been identified.
I’ll discuss why below. Despite their central importance to life as we know it, even the most rudimentary discussion of microorganisms or microbiology (i.e., the study of microorganisms) is rarely included in high school or university curricula.
A Brief History
Tree of Life
Microbes were the first organisms to exist on earth. Our current understanding is that Earth formed 4.6 billion (4,600,000,000) years ago. There is evidence that the first microbes came into existence approximately 3.5 to 3.8 billion years ago (the fossil record indicates that mammals appeared 65 million years ago, and humans showed up a mere 315,000 years ago). Figure 3 illustrates the life on Earth timeline. The bar illustrating Earth’s age is 5 inches (in) long and the one for microorganisms is 4.1 in long. By comparison, the one for plants is 0.2 in and the one for humans is 0.000003 in – too thin to see! Thus, for more than 3 billion years, microbes were the only life forms on Earth.
If we visualize life as a tree, the organisms began to diversify genetically approximately 3.2 to 3.5 billion years ago (Figure 3). This was the time of the last universal common ancestor – LUCA – of all cellular organisms, starting with the bacteria and (thus far) culminating in humans. Before the microbes now classified as members of the kingdom Archaea were discovered near ocean floor, thermal vents in the Marianas Trench, in 1960, the tree of life was thought to have three Kingdoms: Monera (Prokaryota – all single cell organisms that do not have a nucleus or other membrane-bound internal bodies, Protista – all single cell organisms with a nucleus and other membrane-bound bodies, and Eukaryota – all multicellular organisms). As depicted in Figure 3a, in the 1960s through 1990s, Archaea were classified as Archaebacteria. When viewed through a microscope, they appeared to be bacteria. Believing that conditions around Marianas Trench thermal vents was similar to those of primordial Earth, microbiologists initially assumed that Archaea were more ancient than true bacteria – Eubacteriales. As genetic tools became available in the early 1990s, it became apparent that a) Eubacteriales are more ancient than Archaea (Figure 3b), and b) the Archaea are sufficiently distinct genetically to be its own phylogenic Kingdom (phylogenics – the study of evolutionary development and diversification of a species or group of organisms). When Figure 3a was created, fungi were thought to be much more ancient than plants or animals. As Figure 3b illustrates, the phylogenetic tree of life branched off to fungi, plants, and animals a mere half-billion years ago. Thus, fungi are more closely related to us than they are to bacteria.
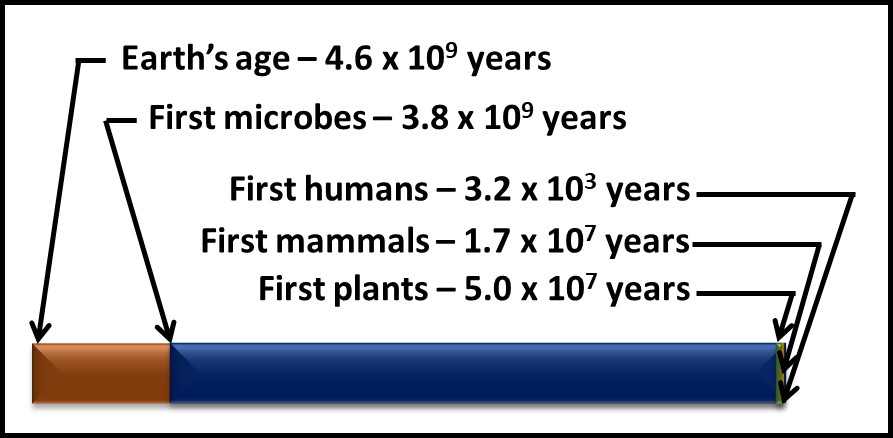
Fig 2. Timelines – microorganisms appeared approximately 1 billion years after Earth was formed and more than 3 billion years before the first plants appeared. Brown bar – Earth’s age; blue bar microorganisms’ age; green bar – time since plants first appeared; yellow bar – time since first animals appeared; purple bar (invisibly narrow line) – time since humans appeared.
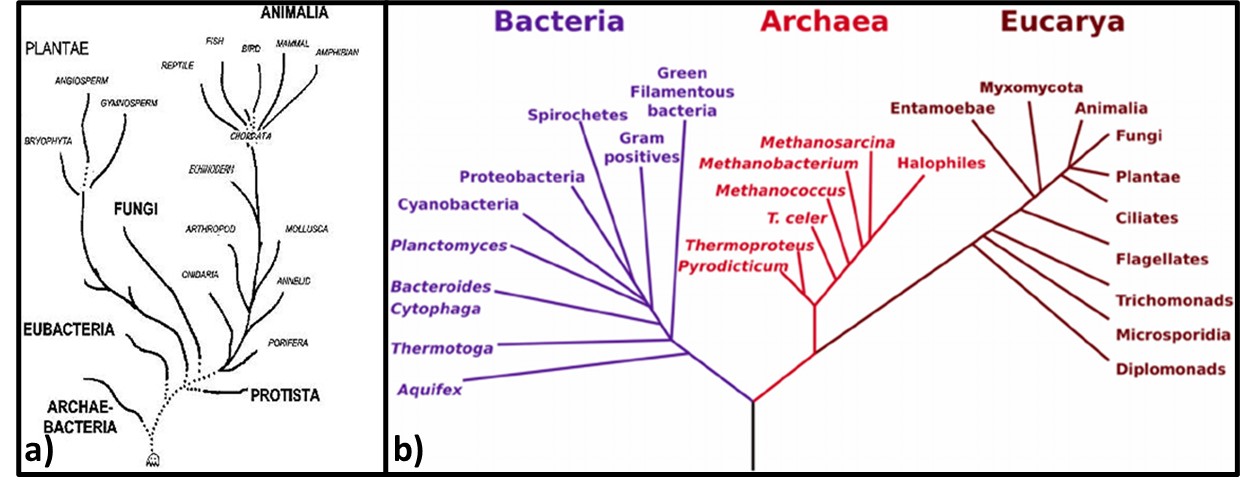
Fig 3. Phylogenic trees – a) Tree from mid-1960s depicting Archaebacteria as being more ancient than Eubacteria; b) Woese et al. (19902) phylogenic tree showing three Kingdoms – Bacteria, Archaea, and Eucarya. Line segment lengths are based on genetic differences (longer lines indicate greater differences). The initial split at bottom center is LUCA.
Genomic testing has been a cottage industry since the late 1990s. A recent diagram (Figure 4) is based on the genomics of 2.3 million different species, from bacteria to humans, illustrates how complex the Tree has become (or as some authors note, the Tree now looks more like a Bush).
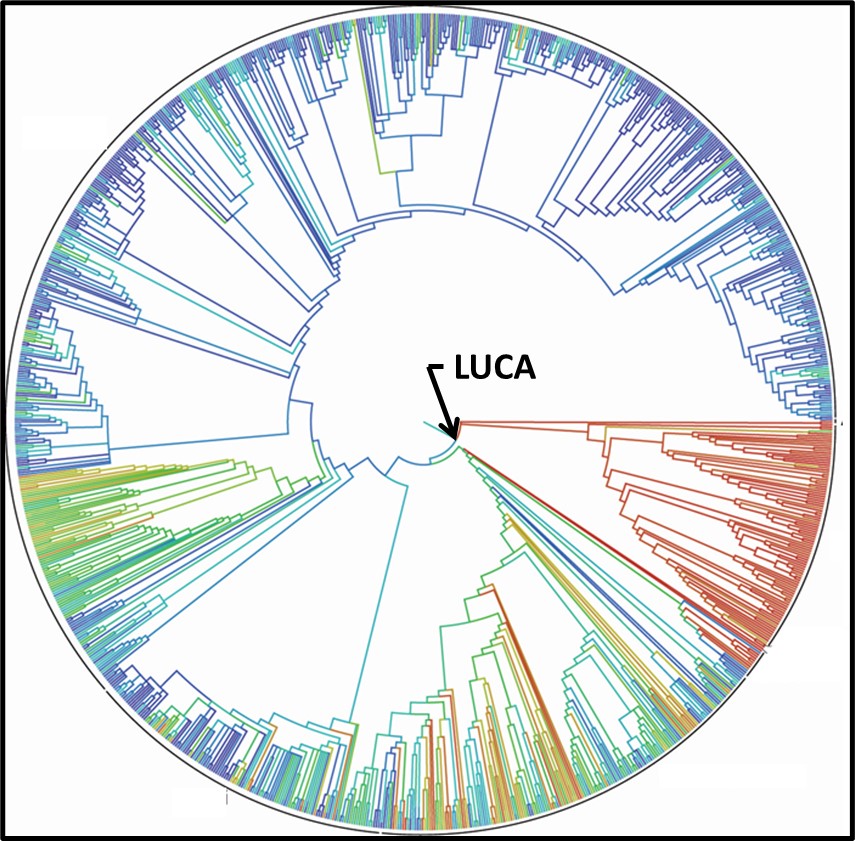
Fig 4. 2015 Tree of Life based on genetic data from 2.3 species.3
Human Awareness
Humans have been using microbes from time immemorial. We have been fermenting grains and grapes, and making cheeses, for as long as we have been cultivating plants or maintaining livestock. Modern microbiology dates from 1665, when Roert Hooke published Micrographia: or some Physiological Descriptions of Minute Bodies made by Magnifying Glasses with Observations and Inquiries Thereupon. A decade later, Antonie van Leeuwenhoek published his observations. Hooke and van Leeuwenhoek had each constructed microscopes through which they observed and created sketches of microorganisms (Figures 5a and 5b). However, it took another 200 years before Louis Pasteur and Robert Koch demonstrated that microbes were living beings and were responsible for fermentation, disease, and spoilage.
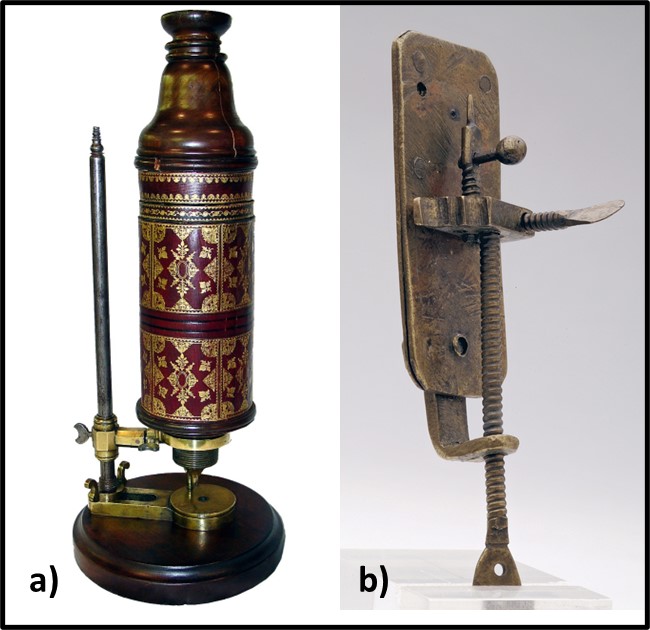
Fig 5. First microscopes – a) replica of Robert Hook’s microscope; b) replica of Antonie van Leeuwenhoek’s microscope.
Louis Pasteur conducted experiments to disprove the theory of spontaneous generation (belief that microbes developed from inanimate components of the materials which they caused to rot) and prove the germ theory of disease (i.e., that microorganisms did not form spontaneously and that they caused disease, biodeterioration, and were the agents responsible for fermentation). Pasture used goosenecked flasks (Figure 6) to demonstrate that microbes did not proliferate (multiply) in broth boiled in the flasks but did in identical flasks that contained unboiled broth. Proliferation also occurred when Pasteur intentionally permitted boiled broth to be exposed to microbially contaminated air (i.e., either by breaking off the gooseneck or tipping the flask). The gooseneck shape allowed air but not microbes to enter the flasks. These experiments led to Pasteur’s development of the pasteurization – the process of heating substances at temperatures sufficient to disinfect but not degrade them.
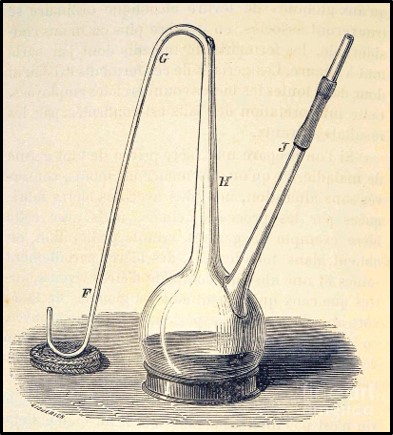
Fig 6. Drawing of Louis Pasteur’s gooseneck flask used to disprove spontaneous generation theory.
While Pasteur was focusing on fermentation microbiology, Robert Koch demonstrated the unequivocal relationship between microbes and disease. Koch demonstrated that the disease, anthrax was caused by the spore forming bacterium, Bacillus anthracis. He also developed the first solid growth media so that he could isolate pure cultures from colonies (Figure 7, zone 5). Pasteur’s and Koch’s research launched the modern age of microbiological research.
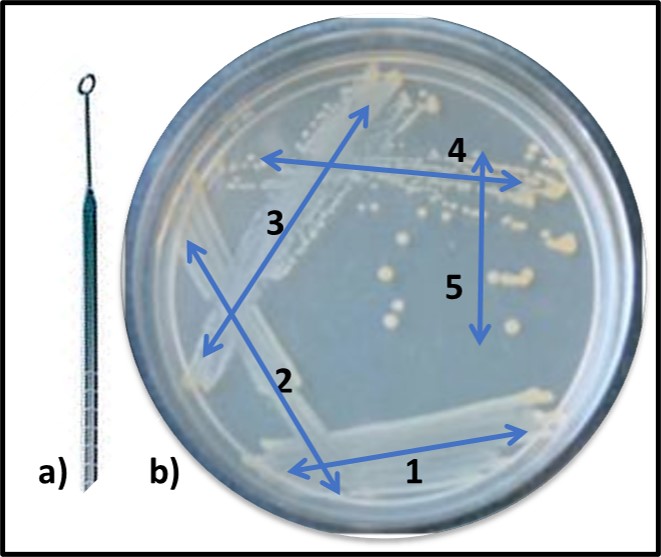
Fig 7. Obtaining a pure (single type of bacterium) culture by the streak plate method – a) specimen is collected using a sterilized inoculating loop; b) successively, initial specimen is deposited onto solid nutrient medium using a back-and forth motion (1), inoculating loop is heat sterilized, cooled, and oved across initial inoculation zone (2). This dilutes the sample. The final iteration (5) typically produces individual colonies after the inoculated plate has been incubated.
As now, much of the research performed during the late 19th century was focused on the relationship between microbes and disease. However, Sergei Winogradsky became the father of microbial ecology. Based on his pioneering research on sulfur metabolism in the late 1880s, Winogradsky developed the theory of biogeochemical cycles. This theory states that elements like sulfur, carbon, nitrogen, and phosphorous cycle through nature (Figure 8). These cycles are primarily mediated by microbial activity. The first paper describing gasoline deterioration by microbes was published in 1895. Starting in the 1920s, considerable effort was focused on oilfield damage caused by microorganisms. This research included the first studies on what was originally called microbially induced corrosion (MIC – now microbiologically influenced corrosion – see MICROBIOLOGICALLY INFLUENCED CORROSION – Biodeterioration Control Associations, Inc. (biodeterioration-control.com)). However, the term biodeterioration was not coined until 1965, when H. J. Hueck offered the definition: “any undesirable change in the properties of a material caused by the vital activities of organisms.”4
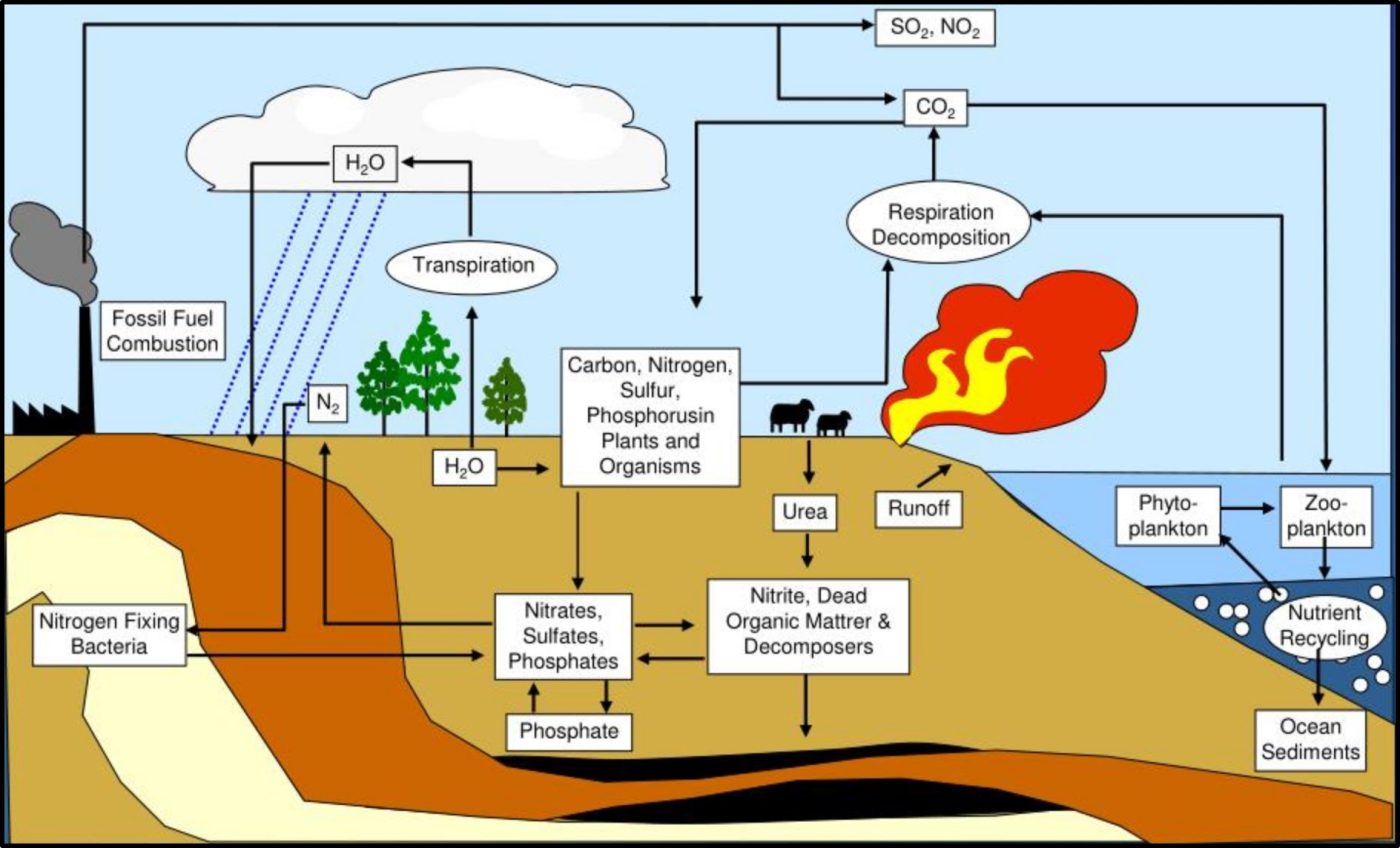
Fig 8. Biogeochemical cycles – this illustration provided a simplified depiction of how carbon (C), nitrogen (N), phosphorous (P), and sulfur (S) cycle through nature.
In my seminars on the topic I explain that biodeterioration and bioremediation are two sides of the same biodegradation coin (Figure 9). As Winogradsky observed, microbes drive biogeochemical cycles. These cycles occur regardless of human intent. When we want biodegradation to occur, we call it bioremediation. When microbial activity causes changes, we’d prefer to prevent, we call it biodeterioration (see https://biodeterioration-control.com/2018/03/).
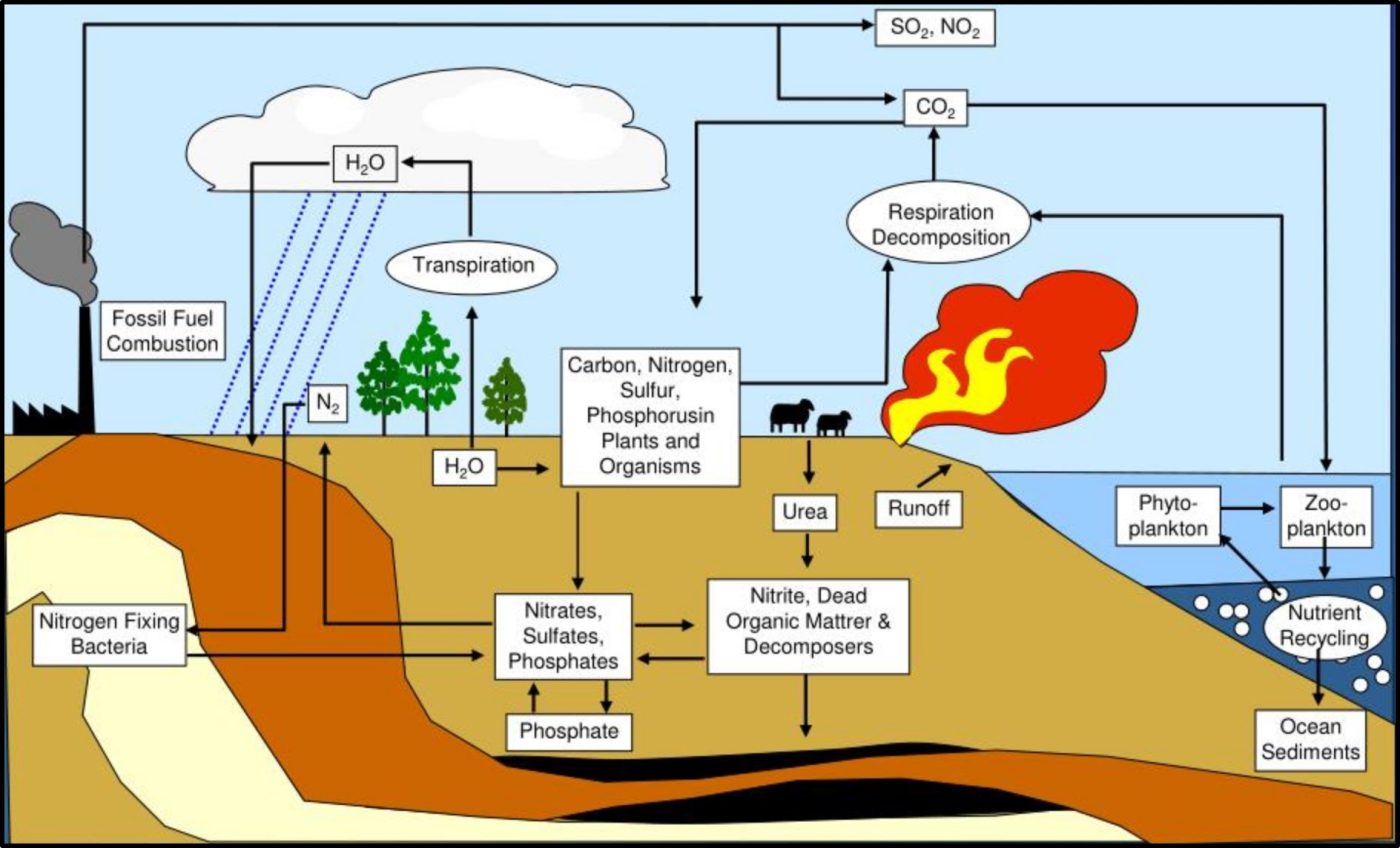
Fig 9. Like the obverse (front) and reverse (back) sides of this 2000 Sacagawea U.S. dollar coin, bioremediation and biodeterioration are flips sides of biodegradation.
Summary
Microbes play invaluable roles in our lives. Our bodies would not function without the microbes that make up he human microbiome. Fewer than 2,000 pathogenic microbes have been identified among the tens of thousands that have been identified and the millions of different types of microbes that exist in nature. Microbes mediate nutrient cycles. This cycling conserves essential nutrients and prevents wastes from accumulating. Biodegradation includes all processes that breakdown organic substances. On one hand, biodegradation is the foundational element of a $0.5 trillion biotechnology industry. On the other, biodeterioration and infectious disease cost $1.5 trillion per year. We are part of the microbial world. To me, that seems like an excellent reason why everyone should have a basic understanding of microbiology.
For more information about fuel system condition monitoring and predictive maintenance, contact me at fredp@biodeterioration-control.com.
1 Bar-On, Y.M, Phillips, R., Milo, R., 2018. The biomass distribution on Earth. Proc Natl Acad Sci U S A., 115(25):6506-6511. https://pubmed.ncbi.nlm.nih.gov/29784790/.
2 Woese, C. R., Kandler, O., Wheelis M.L., 1990. Towards a natural system of organisms: proposal for the domains Archaea, Bacteria, and Eucarya. Proceedings of the National Academy of Sciences of the United States of America. 87 (12): 4576–9. https://doi.org/10.1073/pnas.87.12.4576.
3 Hinchliff, C. et al., 2015. Synthesis of Phylogeny and Taxonomy Into a Comprehensive Tree of Life. Proceedings of the National Academy of Sciences https://www.pnas.org/doi/full/10.1073/pnas.1423041112.
4 Hueck, H.J., 1965. The Biodeterioration of Materials as a Part of Hylobiology. Material und Organismen, 1, 5-34.