MICROBIOLOGY FOR THE UNITINTIATED – PART 3: WHAT IS LIFE – CONTINUED
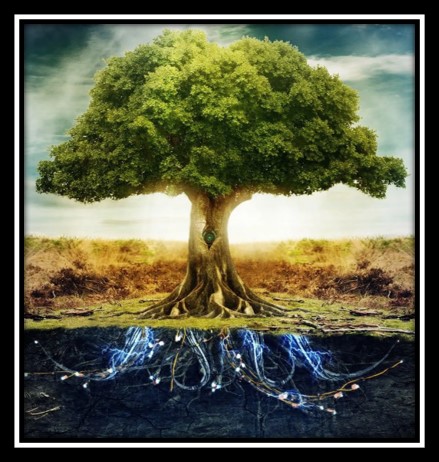
Tree of life
All Organisms Share Common Characteristics
As I explained in last month’s column, all life forms share at least six common properties (variations of this list that include additional properties):
- Order
- Growth
- Homeostasis
- Metabolism
- Reproduction
- Respiration
In April I focused on order and growth. This article will focus on homeostasis and metabolism.
Homeostasis
To survive, cells must be able to regulate their internal environment. Collectively, the numerous processes by which cells maintain a stable internal environment are called homeostasis. For example, cells function optimally when the sodium (Na+) and potassium (K+) concentrations are in balance and at the right levels. Similarly, although some microbes can thrive in environments with pH <2 and others can thrive in environments with pH >12, most microbes maintain an internal pH of approximately 7.2 ± 0.2. Although there are exceptions, conditions inside cells differ from those of their environment. Cell membranes control the movement of solvents (i.e., water) and solutes (dissolved molecules such as Na+, K+, organic molecules used as food, etc.). The membranes function as semipermeable barriers but also contain various molecular structures that actively control the movement of molecules across the barrier. The key point for homeostasis is that, as illustrated in Figure 1, the composition of a cell’s cytoplasm is substantially different from that of its environment.
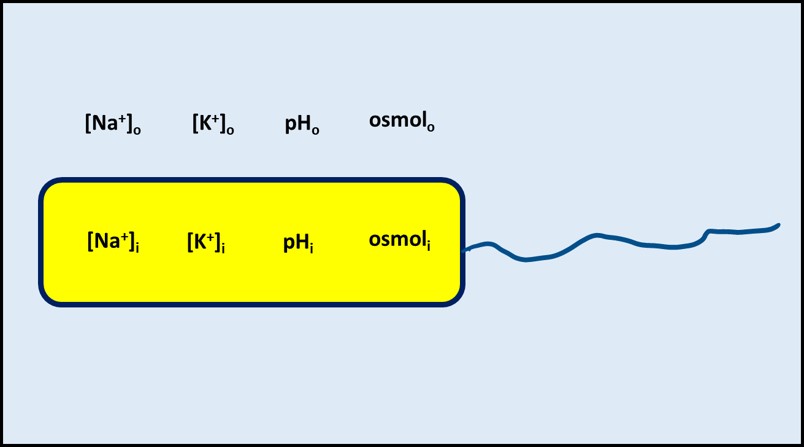
Fig 1. Homeostasis illustrated – solute concentrations inside the cell (e.g., [Na+]I) are maintained at levels needed for healthy metabolism regardless of their concentrations in the external environment (e.g., [Na+]o). Osmol is osmolarity – the total number of moles of solute per L in a solvent (dissolved organic chemicals + cations + anions).
Metabolism
Metabolism includes all the biochemical reactions by which cells obtain energy and convert nutrients into new cell mass, waste products, and heat. Catabolism includes the biochemical reactions that convert large, food molecules (e.g., carbohydrates, proteins, and lipids) into their individual building blocks (e.g., sugars, amino acids, and fatty acids) as illustrated in Figure 2. Anabolism includes the biochemical reactions that assemble building block molecules into new cell components (i.e., carbohydrates, proteins, nucleic acids, lipids, etc.) as shown in Figure 3. Catabolic metabolic pathways typically capture energy and anabolic pathways consume energy. Both types of metabolism generate heat that is lost to the environment in which microbes are growing. Commonly, microbes are classified on how they obtain energy and how they use carbon-based molecules for anabolism.
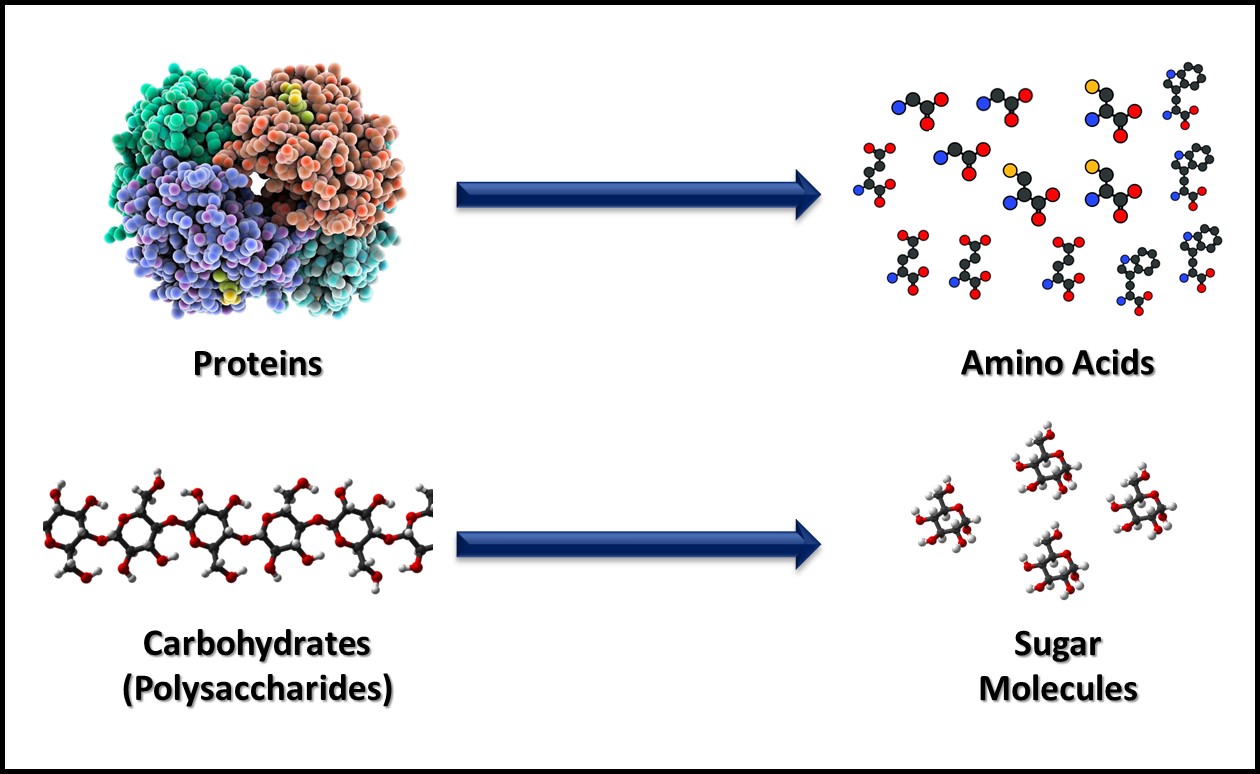
Fig 2. Catabolism – macromolecules are broken down into their building blocks. In this figure, proteins are catabolized to amino acids (top) and carbohydrates are catabolized to individual sugar molecules (bottom).
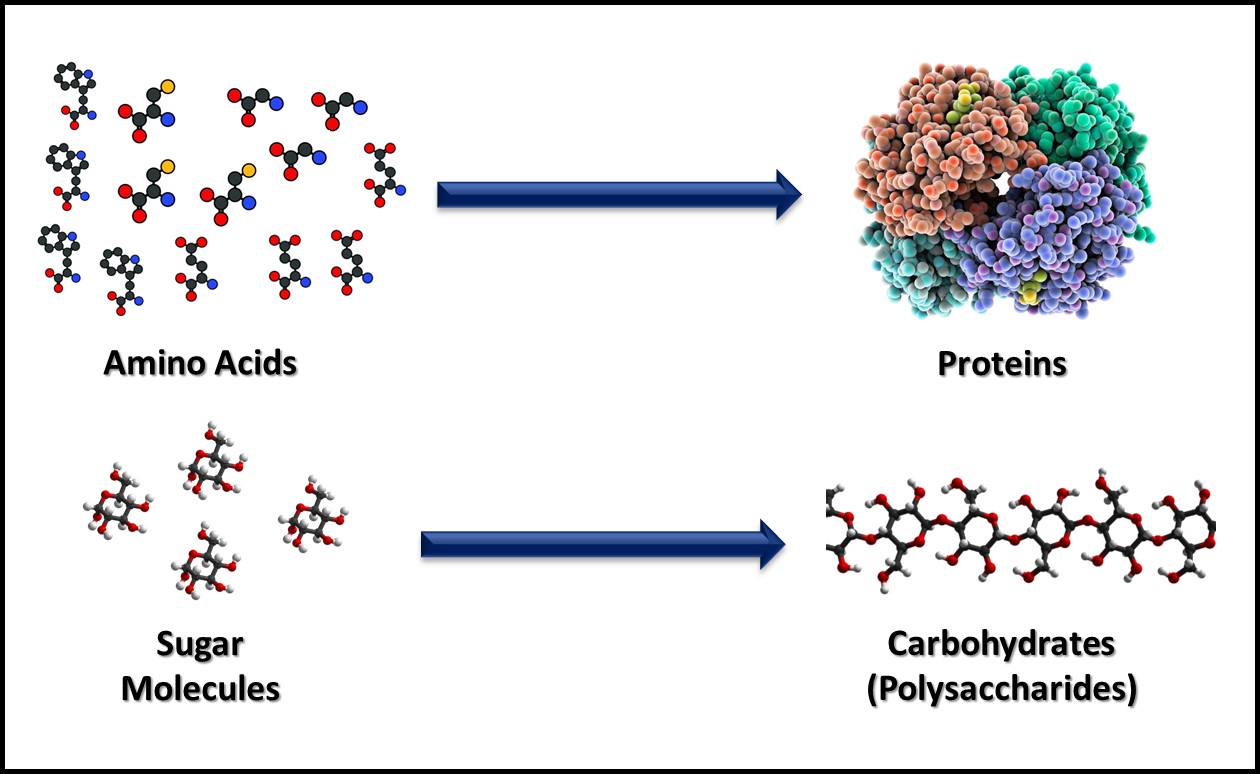
Fig 3. Anabolism – building block molecules such as amino acids (top) and sugars (bottom) are assembled into macromolecules.
Energy metabolism
Phototrophic microorganisms – bacteria and algae – obtain their energy from sunlight. Phototrophic microbes are only likely to cause biodeterioration problems in systems exposed to sunlight.
All other microorganisms are chemotrophs – they conserve energy from chemicals. If the microbe conserves energy by obtaining electrons from inorganic molecules such as ammonium (NH4–), hydrogen (H2), iron (Fe2+), nitrite (NO2–), phosphite (HPO3–), sulfide (HS–), sulfur (S0), among others, it is a lithotroph. If the microbe conserves energy from organic molecules it is a fermenter.
Carbon metabolism
Autotrophic microorganisms use inorganic carbon – i.e., either carbon dioxide (CO2), carbon monoxide (CO), or methane (CH4) – to build organic molecules. Strictly speaking CH4 is a C1 organic compound. However, in the world of microbiology, all three C1 compounds listed here are considered to be inorganic. Thus, photoautotrophs conserve energy from light and use inorganic carbon (CO2). Chemoautotrophs conserve energy from inorganic compounds and use inorganic carbon (CO2, CO, or CH4). Chemoautotrophs are also called chemolithoautotrophs.
Heterotrophic microorganisms use organic molecules (molecules built from carbon and hydrogen, either with or without other atoms such as nitrogen – N, oxygen – O, phosphorus – P, or sulfur – S) as their carbon sources. As noted in the previous paragraph, in the context of microbial metabolism, compounds with two or more carbon atoms are organic. Just like autotrophs, heterotrophs are divided in to two groups, based on their energy source. Photoheterotrophs obtain their energy from light and chemoheterotrophs obtain their energy from organic compounds.
Nutritional requirements vary tremendously among microbes that obtain carbon from organic compounds. Some microbes can only use C2 or C3 molecules such as acetate. Others can attack complex, high molecular weight molecules (macromolecules). Some microbes are fastidious – their diets are severely limited. Others can utilize a variety of organics.
Table 1 summarizes the types of energy and carbon utilization modes used by microorganisms. The possible combinations of energy sources x terminal electron acceptors x carbon sources provide the basis for the microbial world’s tremendous diversity. No single type of microbe has all of the different tools for obtaining energy or food. However, as I explained in May 2022’s What’s New article, microbes most commonly live within biofilm communities.
Table 1. How microorganisms conserve energy and obtain carbon.
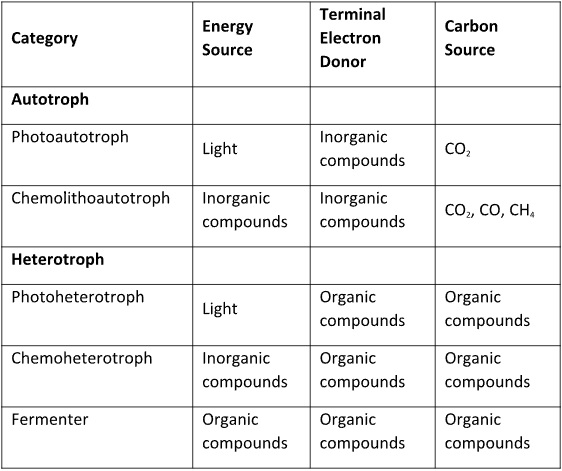
Consortia
Acting in community – consortia – the metabolic capabilities of individual types of microbes create a net capability that far exceeds that of a single type. This is a critical point to understand. Throughout much of the history of environmental microbiology – particularly diagnosis of biodeterioration damage to industrial systems – investigators typically relied on culture test methods to recover and identify the microbes present. Investigators would then attempt to reproduce the biodeterioration process in jars that contained the process fluid (cooling tower water, fuel over water, water-miscible metalworking fluids, etc.). Invariably, it was rarely possible to reproduce the biodeterioration phenomenon. Stakeholders would then speculate that the damage had been caused by “superbugs.” Only after investigators started to use fluids and materials from the systems in which damage was observed did they begin to recognize the power of consortia. The loose analogy here is the difference between one individual and team of tradespeople – carpenters, electricians, plumbers, building a house. It’s the rare individual that can build a modern house. Even a person with all of the required skills will need months or years to complete the work that a crew can finish in a few weeks. Similarly, in industrial systems, biodeterioration damage is caused by different types of microbes – each which a limited number of capabilities working in synergy with the others.
One common dynamic in consortia is the successive degradation of high molecular weight molecules. Consider Figure 4. Some microbes are able to attack fuel molecules. In a closed system, the waste products (metabolites) produced by these microbes accumulates to toxic concentrations Figures 4a. The traditional bacterial growth curve (Figure 4b) reflects the combined effects of nutrient consumption and metabolite toxicity. In consortia, microbes that are unable to eat large molecules eat the metabolites of those that can (Figure 4 c). Additionally, within biofilm environments, aerobic and facultatively anaerobic microbes consume oxygen to create anoxic zones in which obligate anaerobes can thrive (Figure 4d).
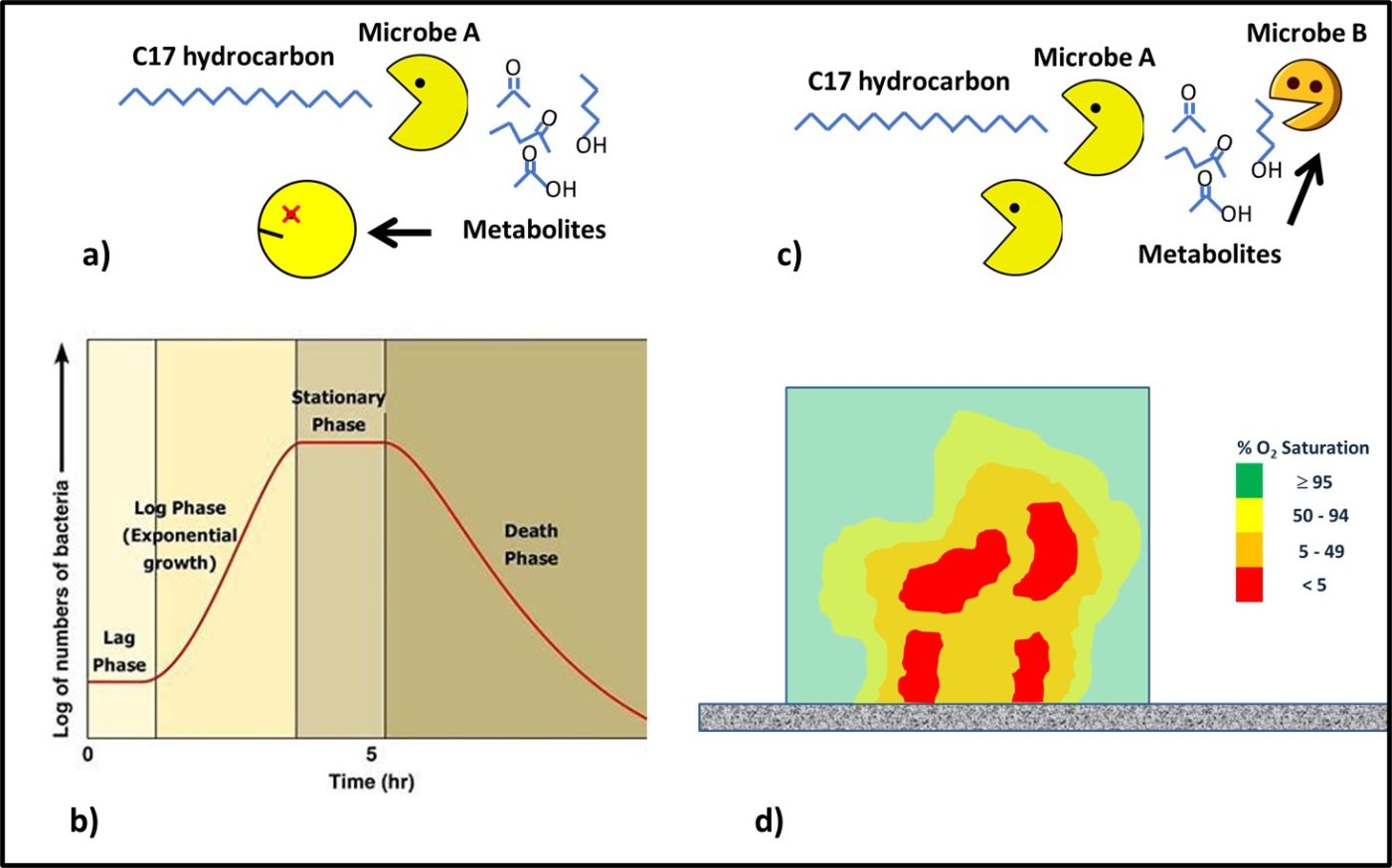
Fig 4. Microbial consortia – a) Microbe A eats C17-hydrocarbons but as metabolites accumulate they become toxic to the microbe; b) traditional closed system growth curve – initially, microbes acclimate to the environment (lag phase); once acclimated the population doubles logarithmically as a function of time (log phase); eventually, as metabolites accumulate, cells die at the same rate new cells are created (stationary phase); ultimately, the die-off rate exceeds the growth rate (death phase); c) Microbe A eats C17-hydrocarbons and Microbe B eats the metabolites – preventing them from accumulating in the closed system; d) oxygen concentration gradient ([O2]) through a biofilm – aerobes at the biofilm-bulk fluid interface (yellow-green) consume oxygen to create an anoxic (red) environment in which anaerobes can thrive.
Summary
Physical and chemical conditions inside microbial cells differ from those of the surrounding environment. Consequently, cells expend substantial energy maintaining constant internal conditions. Collectively, all of the metabolic processes that contribute to maintaining stable internal conditions are called hormonesis. Metabolic processes are either catabolic – when larger molecules are broken down into smaller molecules that are then used as building blocks – or anabolic – the means by which building block molecules are converted into cell components. Additionally, metabolic activity can be divided into energy and carbon metabolism. Energy metabolism involves the use of an energy source and terminal electron donor. Although some microbes can use single carbon (C1) molecules, the vast majority of microbe types that have been identified to date obtain their carbon from organic molecules. Microbes working in consortia do things that no single microbe can.
If you have any questions about this article or microbial contamination-related issues, please contact me at fredp@biodeterioration-control.com.